Dr Victor Luca
12-Jun-21
Unpublished
The views expressed here are solely my own and do not express the views or opinions of any organization with which I am associated.
When it comes to our future climate, environmental and energy challenges it’s easy to bandy about catch-words like sustainability.
Yet, it seems to me, that few people fully appreciate what the word means, or what it really entails, and I include our Climate Change Commission and government policy analysts.
Practically everything we use and make today in the modern world is made from resources that are extracted from the Earth’s surface. That includes your home, car, cell phone, power plant, the food you eat, the clothes you wear, everything.
Disruption in transportation is finally coming through the long overdue replacement of internal combustion engine (ICE) vehicles with either battery electric vehicles (BEV) or fuel cell electric vehicles (FCEV). Promotion of this transition is set to play a central role in many national strategies with the aim of reaching zero emissions by 2050 including us in New Zealand.
How many of you have stopped to wonder what these things that we can’t live without are really made of and where the materials to make them come from? What are the life-cycle green-house gas (GHG) emissions involved in their extraction from Earth’s surface?
Here I want to focus on the amount of natural resources needed to make these things, how much of these materials are actually readily available on the Earth’s surface and how fast we are consuming them?
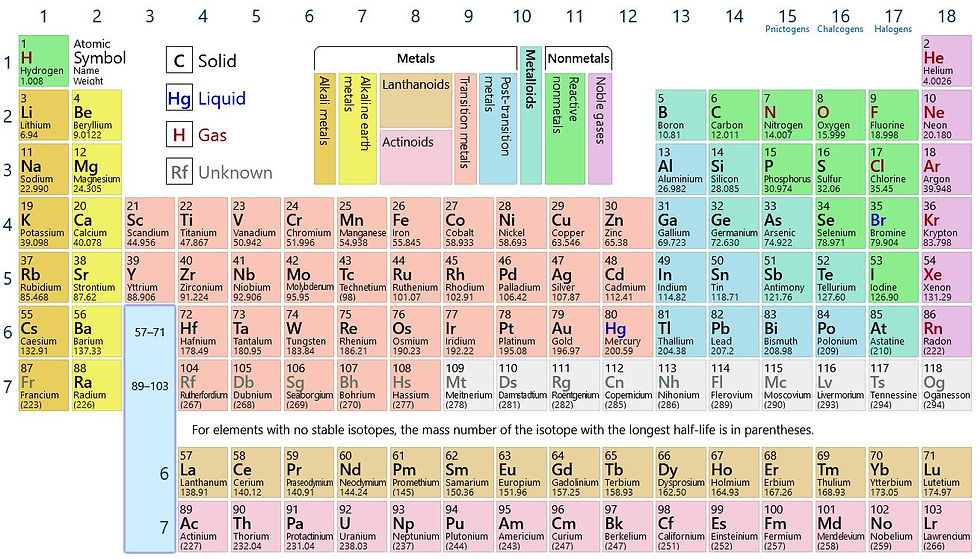
Figure 1. The modern periodic table of elements.
The Russian chemist Dmitri Mendeleev published the first recognizable table of elements in 1869. At the time only 63 elements were known. Chemists and physicists have arranged the elements according to increasing atomic number in a table known as the Periodic Table of Elements. The atomic number is the number of protons in the nucleus of an atom. The atomic structure of elements determines virtually all the chemical properties of the elements.
Since Medeleev’s table, many new elements have been discovered and the periodic table has been gradually filled in. As far as we know today, there are 118 elements in the universe. I am sure many of us are familiar with many of these elements. For example, nearer the top of the table (Figure 1) we have non-metals such as oxygen which is given the symbol O and commonly found as a gas under normal conditions, or carbon from which we are made and which is given the symbol C. Metals like aluminum (Al), iron (Fe), copper (Cu), gold (Au) silver (Ag) which appear in rows 3 - 6 are commonplace.
However, many elements in the periodic table, especially those lower down in the table are probably totally unfamiliar to the majority of readers. Some of elements in the first few rows of the table are going to be critical for the energy transition away from fossil fuels that we so desperately need. They include important materials such as lithium, cobalt, nickel and manganese that are critical for making the lithium-ion batteries used in today’s electric vehicles. The heavy radioactive elements in the last row such as uranium and plutonium are already used to create relatively clean nuclear energy.
The rare earth elements (REE) are metallic elements with funny names numbered 57 through to 71 in the table. These REEs are critical for the production of the powerful magnets used in the traction electric motors that are part of the drive trains of electric cars and wind turbine generators. They are also important in fighter jets and many modern appliances and portable electronics.
The climate change commission has recently told us that we all need to be driving electric vehicles by 2035 to achieve our target of zero emissions by 2050. Similar targets have been set by almost every developed country. But have they thought about how we are going to get there? Do we even have the materials resource base to sustain exponential growth in these things?
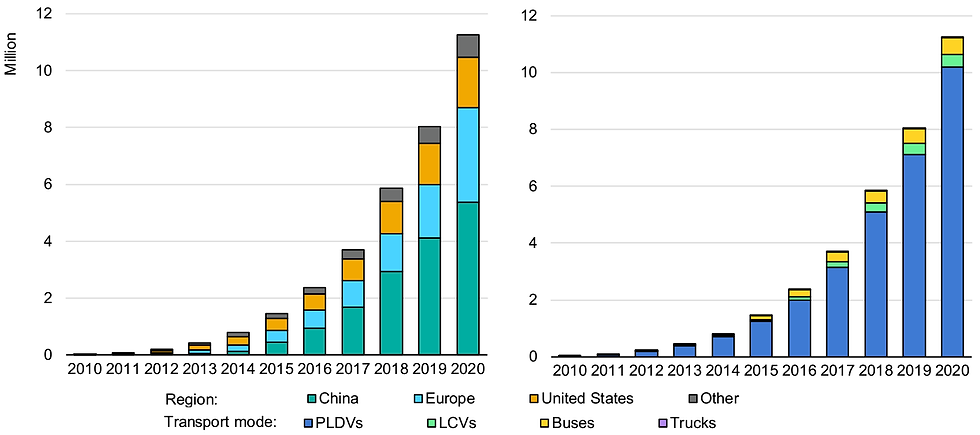
Figure 2. Cumulative car sales. Source: IEA - Global EV Outlook 2021 - Accelerating ambitions despite the pandemic.
Let’s take as an example the electrification of transport which the Climate Change Commission has said we desperately need. Figure 2 shows the cumulative number of BEVs sold each year. It is apparent that we reached a global total of about 11 million BEVs in 2020. It is clear that the growth in sales is exponential (hockey stick curve). Because of the need to electrify transport, and given that the dominant technology involves lithium ion batteries (LIB) and mostly permanent magnet traction motors, the demand for lithium, rare earth elements and copper is also expected to grow exponentially.
You can see from the graph that up to 2020 a total of about 5 million EVs have been sold in China. The Chinese government, and many other governments, have mandated that 25% of the vehicle fleet will be EVs by 2025.
The global production of motor vehicles in 2020 was 78 million of which some 2.5%, or about 2 million, were EVs. You need about 10 Kg of lithium measured as metal for a single BEV battery. That translates to a requirement for 20 million Kg of lithium per year. However, the number of batteries required is increasing rapidly because of their stationary and grid balancing applications and so much more lithium will be needed in the next few decades.
In 2020 the global lithium production was around 82 million Kg and that means that up to 8.2 million batteries could be produced per year. We must also be cognizant of the fact that lithium ion batteries account for only about 40% of present day lithium consumption, the remainder goes to the production of glasses, ceramics and lubricants. In the future it is likely that large quantities of lithium will also be required to fabricate massive batteries for storing renewable energy.
Right now there are currently 1.4 billion motor vehicles in use worldwide. If the entire current world production of lithium were used for BEVs then it would be possible to produce 8.2 million BEVs per year. At that rate it would take 170 years to convert the existing global fleet from ICE vehicles to BEVs. We would have to multiply the annual production by more than 10 to get it done in 17 years or by around 2040. It is estimated that for 2050 we would need the lithium extraction rate to increase by at least 500%. In order to up the rate of production we would need to get many more lithium mines into production. The problem is that at full tilt it takes somewhere between 5 and 10 years to get a lithium mine into production. Another problem is that much of the known lithium reserves are in salt flats (salars) in a region in South America known as the ‘lithium triangle’ which is in the high altitude arid Andean regions of Argentina, Bolivia and Chile. The water availability in these regions is very limited and the ecosystems quite delicate.
Aside from the issue of production rate there is also the problem of the limit in absolute amount of lithium available. There is currently around 18.4 million tonnes of known lithium reserves. Since we are going to need 1.4 million tonnes of lithium to convert the entire existing global ICE fleet, there possibly is enough lithium available. However, we are not counting an increase in fleet size by around 2% per annum or the aforementioned increase in massive batteries for stationary storage and other uses. We should also not lose sight of the fact that population dynamics studies are pointing to an increase in the world’s population by another 4 billion to reach a total of about 11 billion by 2050 so that the global fleet is only going to grow. Of course, if we can master recycling and do it with a low carbon footprint that will help.
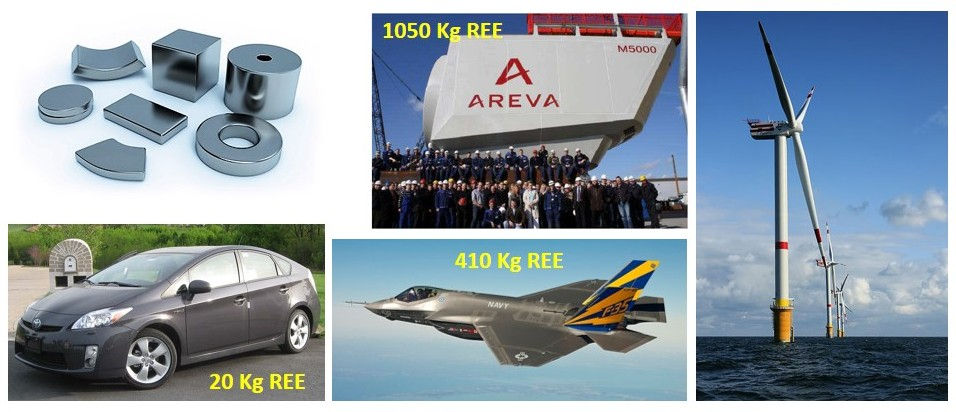
Figure 3. Some current applications of rare earth elements.
In the case of REEs, the situation is even grimmer. REEs are in fact not that rare, but separating them from one another is an extremely complex and energy intensive process. This is one reason why the life cycle emissions of BEVs are far from zero. Certain REEs are used to make the powerful magnets used in the most efficient electric motors and other applications (Figure 3). Other truly rare elements such as indium and tellurium are used in photovoltaic panels and are likely to be in hard to get in the future.
Regardless, there is no reason for not adopting BEVs, because apart from emissions reductions, they are simply better tech. Soon to hit Australian shores, and I presume we will follow, will be nicely designed and extremely well built, cheap (<$35,000 AUD), mid-sized Chinese BEVs like the BYD EA1 with ranges claimed to exceed 600 km and able to do 0 – 100 km/h in 3 - 5 seconds. The arguments to replace obsolete technology based on ICEs for much more sensible EVs is simply too compelling. I keep saying that one day we will laugh at the ICE engine and I am sticking to it.
It should be clear that the challenges that face us as a species are enormous, but the longer we delay taking serious action, the closer to the precipice we move. And those that kid themselves that the problems are not as serious as I have painted them and think that simple panaceas exist, should take their heads out of the sand.
We have only just started the clean energy transition and resource crunches are looming from all directions. Fifty years have now passed since the publication of the famous book Limits to Growth and yet the fundamental message that we were expected to exceed the carrying capacity of Earth by the early 2000s has not sunk in. We should remove the word sustainability from our vocabulary if we don’t understand what it really means.
Комментарии